Patricia Atanelov explores the mechanism by which various antibiotics possess unique antibacterial characteristics that affect S.aureus and E.coli.
Patricia Atanelov
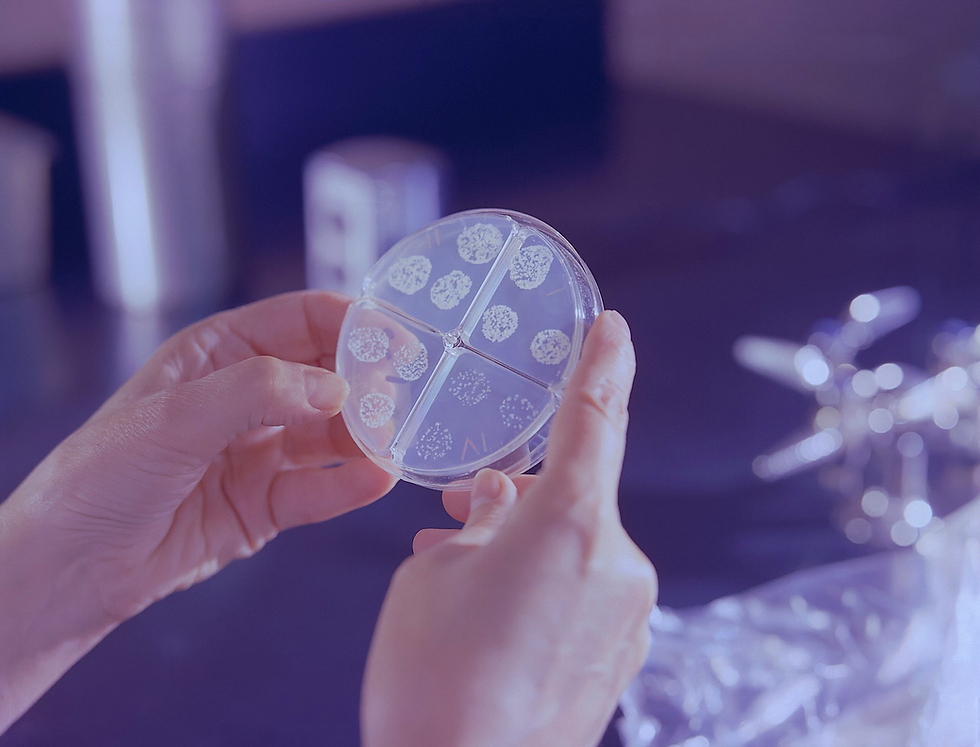
Abstract
Every bacterium is unique in its own way: the environment best fit for its growth, the diverse strains that come from it, its virulence factors, along with what antibiotics will cause the bacteria to form a resistance.
S. aureus and E. coli were both grown on nutrient agar plates and observed to see which antibiotics had the greatest zone of inhibition that would, in turn, show greater efficiency. The primary objective of the experiment was to examine which antibiotic the bacteria had formed a resistance to and to understand how bacterial resistance develops. Antibiotics not only work differently on each bacterium, but they also rely on whether the bacterium is gram-negative or gram-positive. After incubating the bacteria to grow on the nutrient agar plates, the zone of inhibition was measured for each bacterium. Penicillin was the most effective antibiotic for the gram-positive bacterium, S. aureus, with a measurement of 44 mm. Tetracycline had the greatest zone of inhibition, precisely the sole antibiotic that was able to inhibit the E. coli, by 23 mm. Garlic extract was the only antibiotic that was unable to inhibit the bacteria because both S. aureus and E. coli formed a resistance to it, thus having the ability to ultimately kill the antibiotic. Following a thorough analysis of these results, if an organism is infected by either S. aureus or E. coli, it is now known that the most effective antibiotics to consume would be penicillin or tetracycline, as they possess properties that would defeat the virulence factors in the bacteria.
Introduction
Pathogenesis refers to how a disease develops, as well as an organism’s ability to infect (Peterson, 1996). Pathogenic bacteria have a mechanism of action known as virulence factors, which are produced by the organism to create a disease. Specifically, these factors allow the bacteria to multiply in their host or have the ability to spread from one host to another, without being killed. Pathogenic bacteria have a high chance of infection due to their ability to multiply and keep the host alive (Peterson, 1996). The ability of a bacterium to cause a disease correlates to its relative pathogenicity. If a bacterium can cause a disease despite the host’s resistant mechanisms, then it can be considered to have virulence factors (Peterson, 1996).
In 1884, Hans Christian Gram created a technique known as gram staining which allows for the differentiation between gram-positive and gram-negative bacteria through the crystal violet-iodine complex and a safranin counterstain (Coico, 2005). Once the crystal violet-iodine complex is applied to the gram-positive and gram-negative bacteria, the cell wall retains the dye, making both bacteria a purple color. After the treatment of alcohol is applied, the key differences between characteristics of gram-positive and gram-negative bacteria are observed. Gram-positive bacteria appear purple, whereas gram-negative bacteria do not absorb the safranin treatment and instead possess a pink color. Gram-staining allows us to observe morphological features of bacteria such as the characteristic of a peptidoglycan layer. Gram-positive bacteria have a thick and complex layer of peptidoglycan, whereas gram-negative bacteria have a thin layer of peptidoglycan and are resistant to various antibiotics (Chamberlain, 2017). Although different bacteria can lead to infectious diseases, there are modes of treatment that can protect an individual, such as antibiotics.
Antibiotics have the power to not only stop bacteria from multiplying, but to possibly kill it by allowing organisms to naturally defeat the pathogens (Drexler, 2010).
The increase of antibiotic resistance, in turn, increases the effectiveness of the drugs and decreases the development of resistant bacteria. In the experiment, three antibiotics known as penicillin, tetracycline, and garlic extract were used to test the effectiveness of the antibiotic on Staphylococcus aureus and Escherichia coli.
Penicillin can kill bacteria by targeting the transpeptidase which catalyzes the last step in cell wall biosynthesis known as the cross-linking of peptidoglycan (Yocum, et al. 1980). Penicillin is effective against both gram-positive and gram-negative organisms because bacterial resistance to the antibiotic can form due to the diminished permeability of the bacterium’s cell to the penicillin (Soares, et al. 2012). The enzymes that are involved in the transpeptidation reaction react with the B-lactam nucleus, inactivating the transpeptidase and forming peptidoglycan chains. These chains are not linked, meaning they are not as stable. Due to this, the cell wall becomes weaker and ultimately ruptures from an overabundance of fluid in the cell (Yocum, et al. 1980). However, the bacteria can form resistance to penicillin. When the bacteria form a resistance to penicillin, the drug is stopped from reaching the target, decreasing the combative abilities of the bacterial cell toward the antibiotic. By altering the penicillin-binding proteins, a structure that all bacteria possess, it will directly alter the target, forming a resistance to the penicillin (Sprat, 1980).
Tetracycline is an antibiotic that bacteria may become resistant to by changing the ribosomes to prevent binding of the antibiotic or by producing tetracycline-inactivating enzymes. Ultimately, tetracycline inhibits the protein synthesis by altering the ribosomal subunits and preventing the access of tRNA to the acceptor site on the mRNA-ribosome complex. This then prevents the formation of initiating the complex that is required for amino acid protein synthesis (Day, 1966; Suarez, et al. 1965; Maxwell, 1968). In addition to this, it can alter the cytoplasmic membrane which leads to the leakage of nucleotides as well as other complexes in the bacterial cell (Pato, 1977) Like penicillin, bacteria can become resistant to tetracycline through three different mechanisms. Bacteria can limit the access for tetracycline to inhibit ribosomes which prevent the antibiotic from reaching its target. It can also change the ribosome to hinder the binding of tetracycline, which then alters the target. Lastly, the production of tetracycline-inactivating enzymes then inactivates the tetracycline (Goodson, 1994; Roberts, 1996; Speer, et al. 1992).
Furthermore, garlic extract can inhibit the growth of infectious agents, as well as protect organisms from pathogenic bacteria (Abiy, et al. 2016). Specifically, allicin is the antibacterial agent found in garlic and is effective against both gram-positive and gram-negative bacteria. Allicin is produced by a garlic enzyme known as alliinase, from alliin, which has antifungal specificity (Mikaili, et al. 2013). The reasoning behind allicin’s antimicrobial effects is due to its chemical reaction with thiol groups of different enzymes that affect the cysteine proteinase activity (Ankri, et al. 1999). Thus, the cysteine proteinase enzymes can damage and invade tissues, causing infections in organisms.
Staphylococcus aureus is considered a gram-positive bacteria that is responsible for causing various diseases (Taylor, et al. 2020). S. aureus can be located in the environment and on a human’s skin, particularly in the nasal area. Although it is very unlikely that it will affect an individual with healthy skin, it may enter the bloodstream or internal tissues which can lead to serious infections (Lowy, 1998). Most infections can be transmitted through direct contact but some may use other mechanisms (Rasigade, et al. 2014). Treatment for the infection becomes difficult due to the formation of a resistant strain known as MSRA (Methicillin-Resistant Staphylococcus aureus). S. aureus is responsible for the most common bacterial infections in humans and depending on the strain and site of infection, it can cause diseases such as pneumonia, bacteremia, osteomyelitis, etc. (Tong, et al. 2015). The MRSA strain has bacterial resistance to various antibiotics. This is why the infections are caused by pathogens, whether it is hospital or community-acquired (Chambers, 2005; Boucher et al. 2008).
Escherichia coli is a gram-negative bacterium that is a foodborne pathogen known for causing diseases to humans on a global scale (Lim, et al. 2010). Harmless strains of E. coli are found in the gastrointestinal part of humans and animals. However, other strains can become pathogenic bacteria by acquiring factors of a virus through plasmids, bacteriophages, etc. E. coli may be transmitted through the consumption of contaminated water and food, direct contact, or from an animal to a human (Rangel, et al. 2005). E. coli O157:H7 is the cause of many infections which can result in a much more serious disease such as HUS (Hemolytic Uremic Syndrome) or TTP (thrombocytopenic purpura) (Banatvala, et al. 2001).
The objective of the antibiotic experiment conducted in class was to determine which antibiotic (penicillin, tetracycline, or garlic extract) had a stronger antibacterial effect on the bacteria (Staphylococcus aureus & Escherichia coli) by observing the zone of inhibition. The larger the zone of inhibition was, the greater the effect the antibiotic had on the bacteria. Thus, this would conclude whether an antibiotic was effective based on the bacteria’s resistance to it. Since all bacteria have some sort of resistance, this will allow us to see not only which antibiotic is the most effective but also what characteristic about the antibiotic makes it stronger than the others. If Staphylococcus aureus is a gram-positive bacterium and Escherichia coli is a gram-negative bacterium, then penicillin will have the greatest effect on S. aureus, and the tetracycline will be the most effective on E. coli because the antibiotics inhibit the production of the peptidoglycan layer, forming antibacterial resistance properties. However, garlic extract will not affect E. coli or S. aureus because the bacteria will form a resistance to the antibiotic preventing the garlic extract from inhibiting the peptidoglycan layer.
Materials and Methods
To conduct this experiment, certain materials were needed. First, two nutrient agar plates were obtained. One was labeled Staphylococcus aureus and the second plate was labeled Escherichia coli. The bacteria were swabbed on two different plates and isolated to avoid any contamination. The antibiotic disks consisting of penicillin, tetracycline, and garlic extract were placed in each nutrient agar plate with the bacteria. The antibiotics were placed on different ends of the nutrient agar plate to make sure that the zone of inhibition could be easily measured. Next, the nutrient agar plates were placed in an incubator for a total of two days at 37 degrees Celsius which allowed it to grow in an environment best fit for the bacteria. After the two days had passed, the bacteria were taken out of the incubator. The zone of inhibition for each antibiotic on both plates of bacteria were measured in millimeters to determine how effective it was.
Results
Table-Antibiotic Zone of Inhibition vs Bacteria:
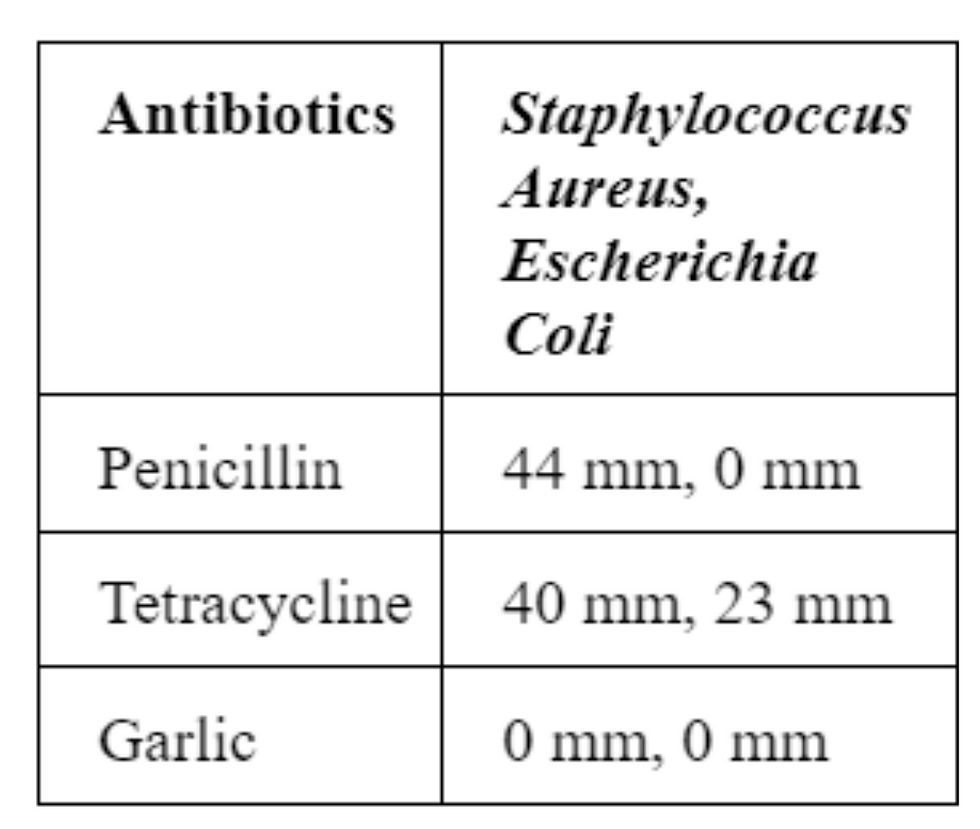
The table above displays the measurements that were taken from observing the zone of inhibition after the antibiotics were placed on each nutrient agar plate with the bacteria. Staphylococcus aureus is a gram-positive bacterium whereas Escherichia coli is a gram-negative bacterium, thus each antibiotic will have a different effect on the bacteria. Analyzing the zone of inhibition of the antibiotics on Staphylococcus aureus, penicillin had the greatest zone of inhibition at 44 mm, meaning that it was the most effective antibiotic. Tetracycline was not as effective as penicillin on S. aureus. However, it did have a large zone of inhibition of 40 mm, meaning it could be considered an effective antibiotic. Garlic extract was not able to inhibit S. aureus or E. coli, meaning that both bacteria developed a form of antibiotic resistance and those antibiotics would not be considered effective.
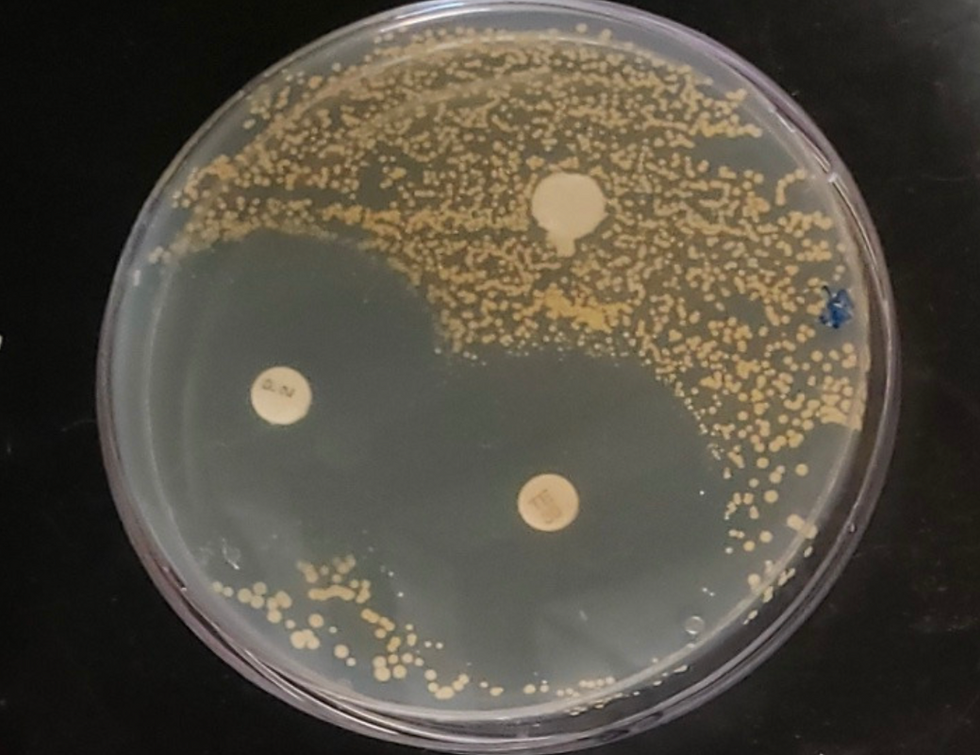
Figure 1 - Antibiotics' Effect on Staphylococcus aureus
On the petri dish above, Staphylococcus aureus was being grown on the medium with three different antibiotics present. Garlic extract was placed at the top of the petri dish, but the bacteria formed a resistance to the garlic extract. Thus, the antibiotic was not able to inhibit S. aureus. Garlic extract had a zone of inhibition of 0 mm, meaning that it would not be considered an effective antibiotic for the bacteria. Penicillin was inserted on the bottom-left hand corner of the petri dish. Ultimately it had the greatest zone of inhibition of 44 mm, meaning that it was the most effective on the S. aureus. Lastly, in the bottom-right corner of the petri dish, tetracycline had a very close zone of inhibition of 40 mm compared to the penicillin. However, it was still not as effective.
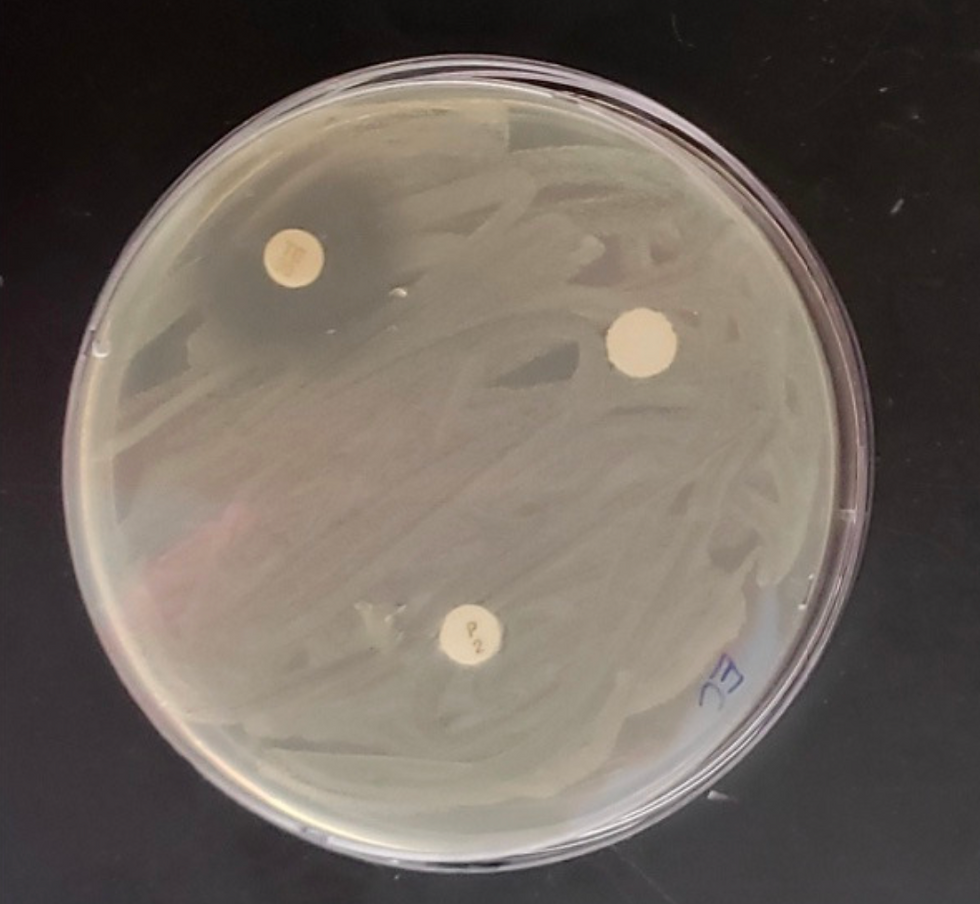
Figure 2 - Antibiotics' Effect on Escherichia coli
On the petri dish, Escherichia coli was being grown on a medium plate with three different antibiotics to determine which were most effective based on their zones of inhibitions. In the top left-hand corner, tetracycline was inserted and it had the largest zone of inhibition at 23 mm. Thus, the E. coli does have antibacterial resistance to the tetracycline because the bacteria will not be able to grow if the antibiotic is present. The penicillin and garlic extract were not able to inhibit the bacteria because E. coli does not possess bacterial resistance to those specific antibiotics. The zone of inhibition for both penicillin and garlic extract were both 0 mm, meaning they are not effective for inhibiting E. coli.
Discussion
Once the bacteria were incubated for two days at their optimal temperature to grow, the effects of the antibiotics were then able to be observed. Penicillin had the greatest zone of inhibition on Staphylococcus aureus because the antibiotic was able to inhibit the bacteria’s peptidoglycan layer, making the cell wall fragile. Due to the inhibition of the cell wall, the bacteria is then unable to grow on the antibiotic without forming a resistance to it. In addition to this, the alteration of penicillin’s binding protein forms a resistance in S. aureus because PBPs are membrane-bound enzymes that can catalyze the transpeptidase reaction, which is important for the cross-linking of peptidoglycan (Hartman, et al. 1984). Thus, the observation and calculation of the zone of inhibition confirm the hypothesized results in that penicillin had a zone of inhibition of 44 mm meaning that it was the most effective antibiotic on S. aureus. On the other hand, tetracycline had the greatest zone of inhibition on Escherichia coli meaning that it was the most effective antibiotic, unlike penicillin and garlic extract, which were not able to inhibit the bacteria. Tetracycline had the greatest effect because it was able to inhibit the protein synthesis in the E. coli, preventing the ribosomal subunits from forming and causing the bacteria to become resistant. This mechanism involves tetracycline binding to the bacteria’s ribosomes, interacting with its ribosomal RNA, and then arresting the translation of the ribosomal subunit which is responsible for protein synthesis (Brodersen, et al. 2000; Maxwell, 1967; Pioletti, et al. 2001 ). Similar to the hypothesized results of penicillin’s effect on S. aureus, the expected results for tetracycline’s effect on the gram-negative bacteria E. coli were also confirmed. Since S. aureus is a gram-positive bacteria, it has a thick peptidoglycan layer, making it less resistant to many antibiotics. However, penicillin can inhibit the synthesis of the peptidoglycan layer, unlike the other antibiotics, which is why it was expected to have the greatest zone of inhibition. Unlike S. aureus, E. coli is a gram-negative bacteria meaning it has a thinner peptidoglycan layer and is more resistant to many antibiotics. Thus, tetracycline is more effective because instead of targeting the peptidoglycan layer, it targets protein synthesis as well as the outer lipid membrane. Since both bacteria possess different characteristics, they differ in the ways that the antibiotics would affect them.
During experimentation, there was room for many potential errors, but they could have been avoided. For example, not incubating the bacteria could lead to an issue where the bacteria wasn’t grown in an optimal environment, possibly changing the way that the antibiotic would affect the bacteria. By not labeling or taking note of the placement of the antibiotic on the nutrient agar plate, it could have switched the results by not having the accurate observation of which antibiotic had the largest zone of inhibition. Another error could include having a faulty nutrient agar plate, such as it being indented, broken, or contaminated with other bacteria, which can cause problems. To avoid such complications during an experiment, certain actions can be taken. For example, by repeating an experiment a minimum of 3 times, not only will it reduce the chances of having a mathematical error or simple overall mistakes, but it will allow the chance to compare results to see if there was a drastic change or if the effects seemed approximately the same in all three trials. In addition to this, it is important to focus on the controlled variables to maintain the accuracy of an experiment. This may include having the same type of nutrient agar plate for both types of bacteria, using the same strain of bacteria, having the bacteria grow at the same temperature, and using the same unit of measurement when recording the data. Overall, in an experiment, there are many areas where an error can occur, some of which may be difficult to avoid, however, controlled variables must be carefully observed as they may cause severe complications in the experiment.
References
1...Abiy, E., & Berhe, A. (2016, November 14). Anti-Bacterial Effect of Garlic (Allium sativum)
against Clinical Isolates of Staphylococcus aureus and Escherichia coli from Patients Attending Hawassa Referral Hospital, Ethiopia. Retrieved from https://infectious-diseases-and-treatment.imedpub.com/antibacterial-effect-of-garlic-allium-sativumagainst-clinical-isolat es-of-staphylococcusaureus-and-escherichia-coli-from-patientsa.php?aid=17777
2. Banatvala, N., Griffin, P. M., Greene, K. D., Barrett, T. J., Bibb, W. F., Green, J. H., Wells, J.
G., & Hemolytic Uremic Syndrome Study Collaborators (2001). The United States National Prospective Hemolytic Uremic Syndrome Study: microbiologic, serologic, clinical, and epidemiologic findings. The Journal of infectious diseases, 183(7), 1063–1070. Retrieved from ology, Appendix 3, .https://doi.org/10.1086/319269
3. Boucher, H. W., & Corey, G. R. (2008). Epidemiology of methicillin-resistant Staphylococcus
aureus. Clinical infectious diseases : an official publication of the Infectious Diseases Society of America, 46 Suppl 5, S344–S349. Retrieved from https://doi.org/10.1086/533590
4. Brodersen, D. E., Clemons, W. M., Jr, Carter, A. P., Morgan-Warren, R. J., Wimberly, B. T., &
Ramakrishnan, V. (2000). The structural basis for the action of the antibiotics
tetracycline, pactamycin, and hygromycin B on the 30S ribosomal subunit. Cell,
103(7), 1143–1154. Retrieved from https://doi.org/10.1016/s0092-8674(00)00216-6
5. Centers for Disease Control and Prevention (CDC) (2003). Outbreaks of community-associated methicillin-resistant Staphylococcus aureus skin infections--Los Angeles County, California, 2002-2003. MMWR. Morbidity and mortality weekly report, 52(5), 88.
6. Chamberlain, N. (2017). Gram staining rules. Retrieved from
https://www.atsu.edu/faculty/chamberlain/mosdoh/gramstainingrules.htm
7. Chambers H. F. (2005). Community-associated MRSA--resistance and virulence converge.
The New England journal of medicine, 352(14), 1485–1487. Retrieved from https://doi.org/10.1056/NEJMe058023
8. Coico R. (2005). Gram staining. Current protocols in microbi
Retrieved from https://doi.org/10.1002/9780471729259.mca03cs00
9. Day L. E. (1966). Tetracycline inhibition of cell-free protein synthesis. II. Effect of the
binding of tetracycline to the components of the system. Journal of bacteriology, 92(1), 197–203. Retrieved from https://doi.org/10.1128/JB.92.1.197-203.1966
10. Drexler M; Institute of Medicine (US). What You Need to Know About Infectious Disease.
Washington (DC): National Academies Press (US); 2010. IV, Prevention and Treatment. Retrieved from: https://www.ncbi.nlm.nih.gov/books/NBK209704/
11. Frost, L. S., Leplae, R., Summers, A. O., & Toussaint, A. (2005). Mobile genetic elements: the agents of open source evolution. Nature reviews. Microbiology, 3(9), 722–732. Retrieved from https://doi.org/10.1038/nrmicro1235
12. Ghuysen J. M. (1994). Molecular structures of penicillin-binding proteins and beta-lactamases. Trends in microbiology, 2(10), 372–380. Retrieved from
https://doi.org/10.1016/0966-842x(94)90614-9
13. Goodson J. M. (1994). Antimicrobial strategies for treatment of periodontal diseases.
Periodontology 2000, 5, 142–168. Retrieved from https://doi.org/10.1111/j.1600-0757.1994.tb00022.x
14. Griffin, P. M., & Tauxe, R. V. (1991). The epidemiology of infections caused by Escherichia
coli O157:H7, other enterohemorrhagic E. coli, and the associated hemolytic uremicsyndrome. Epidemiologic reviews, 13, 60–98. Retrieved from https://doi.org/10.1093/oxfordjournals.epirev.a036079
15. Hartman, B. J., & Tomasz, A. (1984). Low-affinity penicillin-binding protein associated with
beta-lactam resistance in Staphylococcus aureus. Journal of bacteriology, 158(2), 513–516. Retrieved from https://doi.org/10.1128/JB.158.2.513-516.1984
16. Jacewicz, M. S., Acheson, D. W., Binion, D. G., West, G. A., Lincicome, L. L., Fiocchi, C., &
Keusch, G. T. (1999). Responses of human intestinal microvascular endothelial cells to Shiga toxins 1 and 2 and pathogenesis of hemorrhagic colitis. Infection and immunity, 67(3), 1439–1444. Retrieved from https://doi.org/10.1128/IAI.67.3.1439-1444.1999
17. Lim, J. Y., Yoon, J., & Hovde, C. J. (2010). A brief overview of Escherichia coli O157:H7 and its plasmid O157. Journal of microbiology and biotechnology, 20(1), 5–14.
18. Lowy F. D. (2003). Antimicrobial resistance: the example of Staphylococcus aureus. The
Journal of clinical investigation, 111(9), 1265–1273. Retrieved from https://doi.org/10.1172/JCI18535
19. Lowy F. D. (1998). Staphylococcus aureus infections. The New England journal of medicine,
339(8), 520–532. Retrieved from https://doi.org/10.1056/NEJM199808203390806
20. Maxwell I. H. (1967). Partial removal of bound transfer RNA from polysomes engaged in
protein synthesis in vitro after addition of tetracycline. Biochimica et biophysica acta, 138(2), 337–346. Retrieved from https://doi.org/10.1016/0005-2787(67)90494-7
21. Maxwell I. H. (1968). Studies of the binding of tetracycline to ribosomes in vitro. Molecular
pharmacology, 4(1), 25–37.
22. Mikaili, P., Maadirad, S., Moloudizargari, M., Aghajanshakeri, S., & Sarahroodi, S. (2013). Therapeutic uses and pharmacological properties of garlic, shallot, and their biologically active compounds. Iranian journal of basic medical sciences, 16(10), 1031–1048.
23. Mortimer, P. G., & Piddock, L. J. (1993). The accumulation of five antibacterial agents in porin-deficient mutants of Escherichia coli. The Journal of antimicrobial chemotherapy, 32(2), 195–213. Retrieved from https://doi.org/10.1093/jac/32.2.195
24. Pato M. L. (1977). Tetracycline inhibits propagation of deoxyribonucleic acid replication and alters membrane properties. Antimicrobial agents and chemotherapy, 11(2), 318–323. Retrieved from https://doi.org/10.1128/aac.11.2.318
25. Peterson JW. Bacterial Pathogenesis. In: Baron S, editor. Medical Microbiology. 4th edition.
Galveston (TX): University of Texas Medical Branch at Galveston; 1996. Chapter 7. Retrieved from https://www.ncbi.nlm.nih.gov/books/NBK8526/
26. Pioletti, M., Schlünzen, F., Harms, J., Zarivach, R., Glühmann, M., Avila, H., Bashan, A., Bartels, H., Auerbach, T., Jacobi, C., Hartsch, T., Yonath, A., & Franceschi, F. (2001).Crystal structures of complexes of the small ribosomal subunit with tetracycline, edeine
and IF3. The EMBO journal, 20(8), 1829–1839. Retrieved from
https://doi.org/10.1093/emboj/20.8.1829
27. Pugsley, A. P., & Schnaitman, C. A. (1978). Outer membrane proteins of Escherichia coli. VII. Evidence that bacteriophage-directed protein 2 functions as a pore. Journal of bacteriology, 133(3), 1181–1189. Retrieved from
https://doi.org/10.1128/JB.133.3.1181-1189.1978
28. Rangel, J. M., Sparling, P. H., Crowe, C., Griffin, P. M., & Swerdlow, D. L. (2005).
Epidemiology of Escherichia coli O157:H7 outbreaks, United States, 1982-2002. Emerging infectious diseases, 11(4), 603–609. Retrieved from https://doi.org/10.3201/eid1104.040739
29. Rasigade, J. P., & Vandenesch, F. (2014). Staphylococcus aureus: a pathogen with still unresolved issues. Infection, genetics and evolution : journal of molecular epidemiology and evolutionary genetics in infectious diseases, 21, 510–514. Retrieved from
https://doi.org/10.1016/j.meegid.2013.08.018
30. Roberts M. C. (1996). Tetracycline resistance determinants: mechanisms of action,
regulation of expression, genetic mobility, and distribution. FEMS microbiology reviews, 19(1), 1–24. Retrieved from https://doi.org/10.1111/j.1574-6976.1996.tb00251.x
31. Schmidt, H., Henkel, B., & Karch, H. (1997). A gene cluster closely related to type II secretion pathway operons of gram-negative bacteria is located on the large plasmid of enterohemorrhagic Escherichia coli O157 strains. FEMS microbiology letters, 148(2), 265–272. Retrieved from https://doi.org/10.1111/j.1574-6968.1997.tb10299.x
32. Serge Ankri, David Mirelman, Antimicrobial properties of allicin from garlic, Microbes and
Infection, Volume 1, Issue 2, 1999, Pages 125-129,ISSN 1286-4579, Retrieved from https://doi.org/10.1016/S1286-4579(99)80003-3.
33. Soares, G. M., Figueiredo, L. C., Faveri, M., Cortelli, S. C., Duarte, P. M., & Feres, M. (2012). Mechanisms of action of systemic antibiotics used in periodontal treatment and mechanisms of bacterial resistance to these drugs. Journal of applied oral science : revista FOB, 20(3), 295–309. Retrieved from https://doi.org/10.1590/s1678-77572012000300002
34. Speer, B. S., Shoemaker, N. B., & Salyers, A. A. (1992). Bacterial resistance to tetracycline:
mechanisms, transfer, and clinical significance. Clinical microbiology reviews, 5(4), 387–399. Retrieved from https://doi.org/10.1128/cmr.5.4.387
35. Spratt B. G. (1980). Biochemical and genetical approaches to the mechanism of action of penicillin. Philosophical transactions of the Royal Society of London. Series B, Biological sciences, 289(1036), 273–283. Retrieved from https://doi.org/10.1098/rstb.1980.0045
36. Suarez, G., & Nathans, D. (2004, December 07). Inhibition of aminoacyl-sRNA binding to ribosomes by tetracycline. Retrieved from https://www.sciencedirect.com/science/article/abs/pii/0006291X6590848X
37. Taylor TA, Unakal CG. Staphylococcus Aureus. [Updated 2020 Aug 23]. In: StatPearls
[Internet]. Treasure Island (FL): StatPearls Publishing; 2021 Jan-. Retrieved from: https://www.ncbi.nlm.nih.gov/books/NBK441868/
38. Thanassi, D. G., Suh, G. S., & Nikaido, H. (1995). Role of outer membrane barrier in efflux-mediated tetracycline resistance of Escherichia coli. Journal of bacteriology, 177(4), 998–1007. Retrieved from https://doi.org/10.1128/jb.177.4.998-1007.1995
39. Tong, S. Y., Davis, J. S., Eichenberger, E., Holland, T. L., & Fowler, V. G., Jr (2015). Staphylococcus aureus infections: epidemiology, pathophysiology, clinical manifestations, and management. Clinical microbiology reviews, 28(3), 603–661. Retrieved from https://doi.org/10.1128/CMR.00134-14
40. Yocum, R. R., Rasmussen, J. R., & Strominger, J. L. (1980). The mechanism of action of penicillin. Penicillin acylates the active site of Bacillus stearothermophilus D-alanine carboxypeptidase. The Journal of biological chemistry, 255(9), 3977–3986.
41. Zhang, H. Z., Hackbarth, C. J., Chansky, K. M., & Chambers, H. F. (2001). A proteolytic transmembrane signaling pathway and resistance to beta-lactams in staphylococci. Science (New York, N.Y.), 291(5510), 1962–1965. Retrieved from https://doi.org/10.1126/science.1055144
Bình luận